One of the questions I’ve been asked regularly since COVID first emerged is whether it is – or will become – a ‘winter virus’. In media coverage, it often feels like there’s an inevitability to the term, as if the timing of epidemic waves is somehow a diary choice on the part of the virus: ‘OK, March is here, time to pack up for the rest of the year’.
In spring 2020, after dramatic reductions in social interactions brought down COVID transmission, some medical commentators confidently claimed the disease must be seasonal, because they’d never seen a respiratory virus spreading outside of winter. Which was surprising, given the 2009 influenza pandemic took off during spring in the North Hemisphere, with the UK epidemic growing larger and larger through May, June and July. The first wave only declined when school holidays started and contact rates among children – who were more susceptible to the new flu virus than adults who’d previously seen similar viruses – dropped for a few weeks.
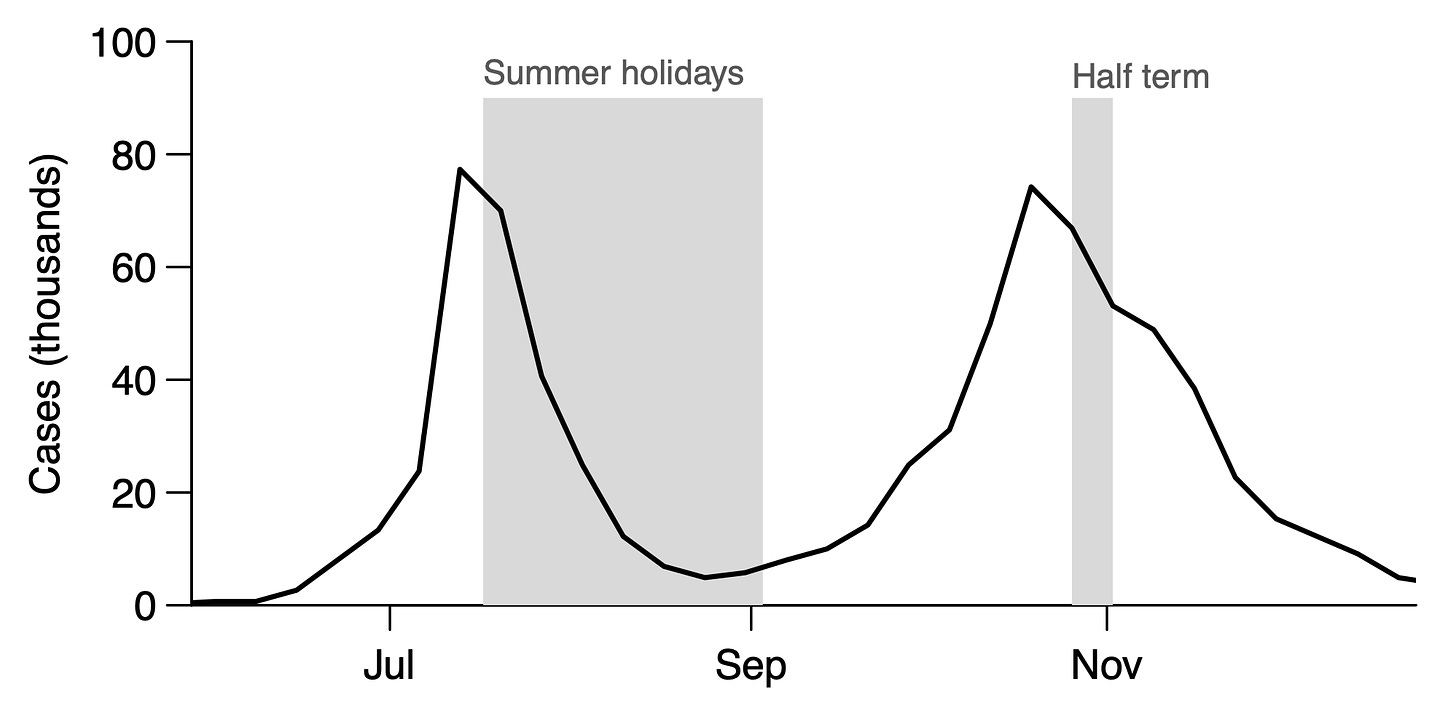
When it comes to COVID, we’ve so far had peaks of infection in the UK in spring 2020, autumn 2020, winter 2020/21, summer 2021, autumn 2021, winter 2021/22, spring 2022, summer 2022, autumn 2022, winter 2022/23, and so on. So it’s been ‘seasonal’ only in the sense that it’s caused epidemics in every single season:
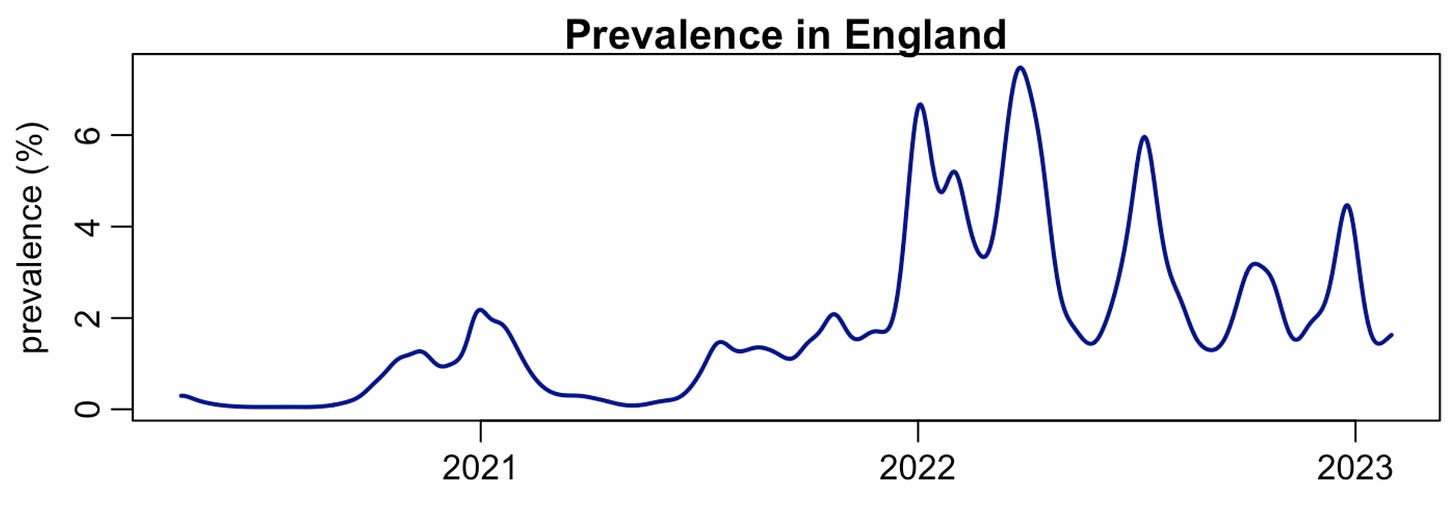
What drives seasonal transmission?
If familiar ‘winter viruses’ only spread at a specific time of year, it’s not by magic. There will be a combination of biological and epidemiological factors behind the patterns we observe. And if that combination of factors changes, we shouldn’t necessarily expect epidemics at the same time of year.
We can quantify changes in transmission using the reproduction number, R. This, as you probably know well by now, is defined as the average number of new people that a typical case infects. If R is above 1, we’d expect the epidemic to grow; if it’s below 1, we’d expect it to decline.
So what can influence R? In The Rules of Contagion, I explain how we can break it down into four main factors. First, transmission depends on the duration a person is infectious for. Then, it depends on how interactions they have during this period, i.e. how many opportunities there are to infect others. Next, it depends on the probability of transmission during these opporunities. And finally, it depends on the probability that the people they meet are susceptible to becoming infected themselves.
I call these four factors the ‘DOTS’: duration, opportunities, transmission probability and susceptibility. If we multiply them together, we get the reproduction number:
We can use this breakdown to understand what can influence the timing of traditional ‘seasonal’ epidemics. If people have more social interactions at festival parties or family gatherings it will drive up opportunities (‘O’). If more interactions are indoors, or the virus can survive longer in certain climate conditions, it can increase the transmission probability (‘T’). And if the winter climate affects baseline levels of immunity, it will increase susceptiblity (‘S’). In other words, multiple factors can potentially contribute to a higher R at certain times of year.
But how much difference will this make in practice? Suppose, hypothetically, we have an infection that has an R value of 1.2 coming into winter, as is the case for some seasonal influenza viruses, and seasonal factors hypothetically combine to make transmission 25% higher in winter than in summer. In early winter, R would therefore be 1.2 and we’ll get epidemic that takes off (because R is above 1). But in summer, we’d have R = 1.2/(1+0.25) = 0.96 and the infection would fail to cause a large epidemic.
This won’t always be the case. If lots of people are susceptible, as they were at the start of the COVID pandemic, then the baseline value of R will be much larger than 1. So seasonal effects won’t be the different between no epidemic and an epidemic; they’ll be the difference between a large epidemic and a slightly larger epidemic.
In May 2020, Rachel Baker and colleagues analysed the difference between seasonal coronaviruses and COVID, suggesting that susceptibility dominated over other factors for COVID:
Levels of susceptibility among the population remain the driving factor for the pandemic, and without effective control measures, the pandemic will persist in the coming months, causing severe outbreaks even in humid climates. Summer will not substantially limit pandemic growth.
The initial R value for the 2009 flu pandemic was lower than for COVID early on. But it still wasn’t that low. Although R declined over the summer in the UK because school holidays started – and hence opportunities for transmission declined – it didn’t stop another wave in autumn as contact patterns among children resumed.
Sometimes even supposedly ‘winter infections’ can do unexpected things when the factors driving transmission break from a stable pattern. Take the 2019 influenza epidemic in Australia, which was unusually early and large:
When Ian Barr and colleagues assessed possible explanations for the epidemic, they concluded it was ‘likely to be a complex mix of climatic, virological and host immunity-related factors’. As we’ve already seen, each of these could affect the DOTS in different ways.
Is winter coming for COVID?
In the context of COVID transmission, suscepibility has so far overpowered seasonal effects. Since the original virus emerged in late 2019, we’ve seen a series of variants that have each evaded prior immunity against infection, allowing them to take hold at seemingly arbitrary points throughout year (you can read more about COVID evolution in my earlier post).
If this pace of evolution continues for COVID, we could continue to see multiple epidemics each year, with the timing of outbreaks dictated more by the randomeness of viral mutation and variant emergence than any predictable seasonal fluctuations in climate or behaviour. But perhaps, as repeat COVID infections and vaccinations – and hence immunity against a range of variants – accumulate in populations globally, the potential for variant emergence will become more constrained. After all, there’s evidence that new variants of familiar seasonal coronaviruses emerge at a slower rate than new variants of seasonal flu viruses.
We may already be seeing some signals that COVID, while not a winter-only virus, is better at causing larger epidemics during the winter period. Let’s look again at that ONS infection prevalence graph. Although COVID epidemics occur throughout the year, the winter/spring peaks tend to be larger than the others nearby:
All things being equal, variants that emerge during winter seem to spread more successfully than those that emerge at other times of year (even if these other variants still cause relatively large waves of infection). We’d need more data points to be confident this is not just chance, but if it is a genuine trend, perhaps we’ll end up seeing a pattern of multiple COVID waves per year, with larger ones during winter, when various factors combine to boost the components of the DOTS, and hence the reproduction number.
That also raises the question of how these winter waves will compete with other seasonal infections. For other infections, researchers have observed ‘viral interference’, whereby infection with one virus triggers an innate immune response that in the short-term makes it harder to get others. They have also observed ‘ecological interference’, where people staying home ill with one virus can’t go out and get infected with another. It’s not clear these effects are large enough to stop COVID finding a winter niche, or to displace other winter infections; we saw peaks of both COVID and influenza in the UK in December 2022. But that doesn’t necessarily mean future years will be the same.
The 2009 influenza pandemic took a couple of years to transition into a more seasonal pattern, with the underlying H1N1 virus continuing to evolve and cause winter epidemics, alongside the H3N2 and B subtypes that have been circulating for longer:
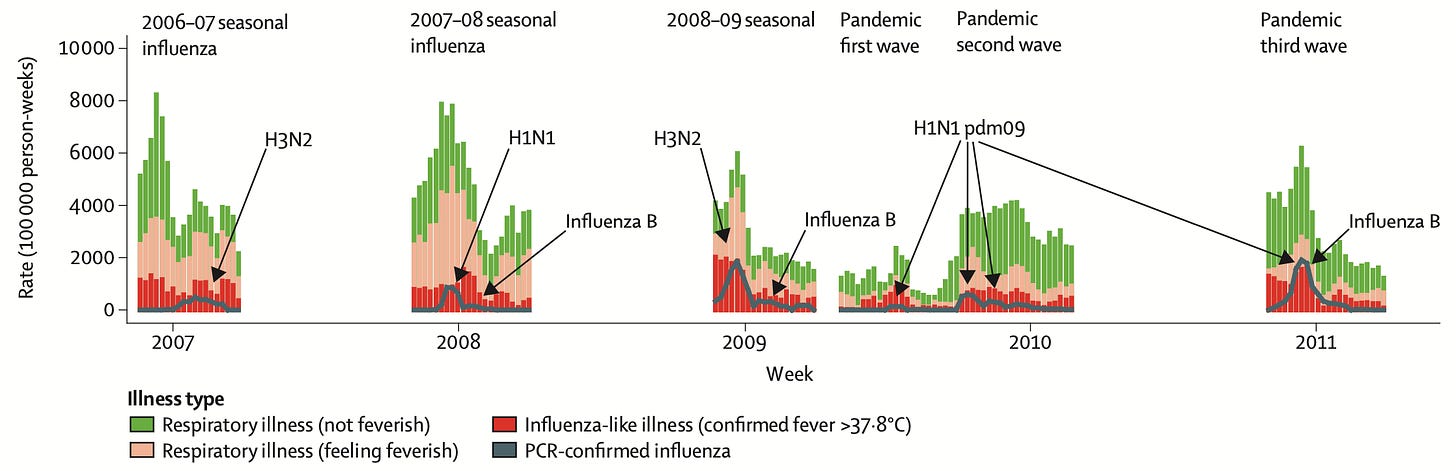
We are still in the early stages of the COVID era, so it will be important to continue to investigate how different factors combine to drive (or not drive) epidemic waves. If we want to understand the dynamics of COVID over the coming years, we’ll need more analysis of data, dynamics and DOTS – and fewer simplistic boxes marked ‘winter virus’.
“ Take the 2019 influenza epidemic in Australia, which was usually early and large:” - shouldn’t that be “unusually early” ?
One minor correction, SARS-CoV-2 is the virus, Covid-19 is the disease.